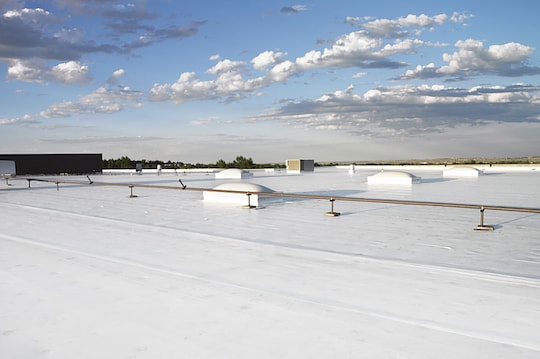
Science du bâtiment
IBC et FM – Quelle est la différence en ce qui concerne la conception tenant compte de la résistance au vent?
IntroductionWind design of roof systems can be confusing from an engineering perspective. La conception qui tient compte de la résistance au vent peut aussi être déroutante en raison des exigences particulières de l'International Building Code (IBC) et de Factory Mutual (FM). Si l'on respecte les exigences de FM, celles de l'IBC doivent-elles aussi être respectées? Quelle est la stratégie de conception qui tient compte de la résistance au vent pour les bâtiments assurés par FM et pour ceux qui ne le sont pas? This blog will discuss the following:IBC is a model code; FM is an insurance companyCompliance with the local building code is a legal requirement; FM is elective (a building owner has the ability to select their insurance carrier)IBC references the ASCE 7 standard; FM provides wind-design methodology via the Ratings Calculator and Assembly Search functions within RoofNavFM-insured buildings must comply with both the IBC and FM requirementsSpecifying "FM" could trigger the "FM process" unknowingly for non-FM insured buildingsWhat is the issue?In roofing specifications, architects have been referencing Factory Mutual (FM) for many decades, especially when it comes to wind design of commercial roofing systems. "Meet FM requirements," "Provide a 1-90 roof system," or just simply "Meet FM" are phrases inserted into specifications. Do these phrases supplant the need to follow the wind-design requirement of the International Building Code (IBC)? (Spoiler alert: The answer is a resounding "NO".)The BasicsThe IBC is a model code, developed by the International Code Council. A model code, such as the IBC, is intended to be adopted by municipalities (e.g., state, city) as the locally enforced building code. The model code can be adopted as-is, or with language removed, with language added, or both. The local building code is enforced through local building code officials. And, very importantly, meeting the local building code is a legal requirement and there can be ramifications when the local building code is not met.Commercial buildings are required to meet the IBC as adopted and amended by the local jurisdiction. For wind design, the IBC requires a roof system be designed based on ASCE 7, Minimum Design Loads for Buildings and Other Structures. (More on the specifics later.)FM Global is an insurance company and a purveyor of design and installation documents for roof systems (e.g., Loss Prevention Data Sheets 1-28, Wind Design). FM Approvals is a testing facility, a third-party certification body, and a developer of Approval Standards (e.g., FM 4470, Single-Ply, Polymer-Modified Bitumen Sheet, Built-Up Roof (BUR) and Liquid Applied Roof Assemblies for use in Class 1 and Noncombustible Roof Deck Construction). FM Approvals also maintains RoofNav (www.RoofNav.com), which provides access to FM Approved roofing systems and related installation recommendations from FM Global. RoofNav is likely the FM tool that is frequently used by architects and roof system designers who are searching for and selecting approved roof systems. This is why many architects and specifiers include some reference to FM in roof system specifications.IBC Wind Design MethodModel building codes, such as the 2018 IBC, when adopted by a local jurisdiction, become the legal requirements for construction. The IBC specifically states, "The I-Codes, including this International Building Code, are used in a variety of ways in both the public and private sectors. Most industry professionals are familiar with the I-Codes as the basis of laws and regulations in communities across the U.S. and in other countries." A more succinct way of stating this is-the local building code is the law.Within the IBC, the building code requirements for roofing and rooftop construction are found in Chapter 15, Roof Assemblies and Rooftop Structures. Section 1501.1, Scope, states "The provisions of this chapter shall govern the design, materials, construction and quality of roof assemblies, and rooftop structures." Wind resistance of roof systems is included in Section 1504, Performance Requirements, and Section 1504.1, Wind resistance of roofs, requires roofs be designed for wind loads according to Chapter 16, Structural Design.Section 1609, Wind Loads, incorporates by reference the standards set forth in ASCE 7; this section includes the following language."1609.1.1 Determination of wind loads. Wind loads on every building or structure shall be determined in accordance with Chapters 26 to 30 of ASCE 7."It's worth noting that the version (i.e., year of publication) of ASCE 7 is not specified in the body of the code; versions of referenced standards are found in Chapter 35.The key point is that the IBC directs users to ASCE 7 to determine design wind pressures (DWP) for roof systems.To continue a bit deeper into the 2018 IBC, Section 1 504,3 is the directive to designers to design roofs to resist design wind pressures."1 504,3 Wind resistance of non ballasted roofs. Roof coverings installed on roofs in accordance with Section 1507 that are mechanically attached or adhered to the roof deck shall be designed to resist the design wind load pressures for components and cladding in accordance with section 1609.5.2."It's important to recognize that Section 1 504,3 specifically ties the wind design of nonballasted roofs to ASCE 7 by referencing a subsection of Section 1609.Additionally, Section 1504.3.1 is the directive to manufacturers to test roof systems to determine wind uplift capacity."1504.3.1 Other roof systems. Built-up, modified bitumen, fully adhered or mechanically attached single-ply roof systems, metal panel roof systems applied to a solid or closely fitted deck and other types of membrane roof coverings shall be tested in accordance with FM 4474, UL 580 or UL 1897."This section provides 3 code-approved test methods to choose from to perform wind-uplift-capacity testing.FM 4474, American National Standard for Evaluation of Simulated Wind Uplift Resistance of Roof Assemblies Using Static Positive and/or Negative Differential PressuresUL 580, Standard for Tests for Uplift Resistance of Roof AssembliesUL 1897, Standard for Uplift Tests for Roof Covering SystemsThese tested systems are found in Approval Listings from organizations like FM, UL, and SPRI. These two videos provide more information about FM Approval's RoofNav and SPRI's Directory of Roofing Assemblies.What's NOT Stated in the IBCNothing in the model code sections referenced here or any other related model code sections within IBC contains a provision that allows a wind-design method other than ASCE 7 to be used. In other words, using FM's RoofNav for wind design of roof systems is not a replacement for following building code requirements that mandate the use of ASCE 7. Of course, designers should always check with the specific requirements of the local building code to determine if the use of FM's RoofNav is allowed for code compliance.FMWe've established that FM Global is an insurance company that provides installation recommendations and FM Approvals provides design information, as well as FM-approved listings. In order to receive an FM Approval Listing, a roof system must be tested in accordance with FM 4470, Single-Ply, Polymer-Modified Bitumen Sheet, Built-Up Roof (BUR) and Liquid Applied Roof Assemblies for use in Class 1 and Noncombustible Roof Deck Construction.FM 4470 includes a battery of tests intended to help determine the long-term performance of a roof system (clearly an important issue for an insurance carrier!).FM 4470 includes the following mandatory tests to be performed:Combustibility (from above and below the roof deck)Wind uplift (FM 4474 is the test method used to determine wind uplift capacity)Hail resistanceWater leakageFoot trafficCorrosionSusceptibility to heat damageFM 4470 also includes requirements for a manufacturer's in-house quality control program that includes an audit program, field inspections during installation, and additional manufacturer responsibilities if products' construction or components are revised.The use of FM 4470 results in a roof system with a "1-60" or "1-75" listing, for example. The "1" represents the roof system is Class 1 for fire resistance (combustibility) from below the deck. The second (e.g., 60, 75) represents the wind-uplift capacity (in pounds per square foot) of the roof system.It is important to recognize that FM 4470 is not listed as one of the test methods for wind-uplift capacity in the 2018 IBC, which means the IBC does not require a roof system to be FM-approved!FM 4474, American National Standard for Evaluation of Simulated Wind Uplift Resistance of Roof Assemblies Using Static Positive and/or Negative Differential Pressures, is a test method to determine wind uplift capacity of roof systems. As noted previously, FM 4474 is the wind-uplift test method that is required to be used within FM 4470 for an FM Approval Listing.FM-insured buildingsBuildings that are FM insured are commonly required by FM to use a roof system that has an FM Approval Listing. More specifically, roof systems intended to be used on FM-insured buildings should use RoofNav to determine wind loads (via the RoofNav Ratings Calculator) and find Approved roof systems (via the RoofNav Assembly Search).Non FM-insured BuildingsTo broadly say "Meet FM" or "Meet FM requirements" in a spec could be interpreted to mean-for non-FM insured buildings-that the wind-design process, deck securement, and roof system installation should follow ALL of the specific FM processes and recommendations that are used for FM-insured buildings.As the architect or specifier working on a building that is not insured by FM, is the vague specification language truly intended to bring the entire "FM process" into the wind design and installation of a roof system? Probably not. It is more likely the vague specification language referencing FM is intended to be a way to state that the assembly must meet local building code requirements for wind design.Saying "Meet FM" or "Meet FM requirements" does not preempt or override the requirements of the IBC, as adopted by local building code, that are legally required to be performed by the Architect of Record when it comes to wind design of roof systems. (Additional information about code requirements for wind design can be found here.)ConclusionThe IBC, as adopted by local building codes, is required by law and references ASCE 7 as the standard to be used for determining design wind pressures for roof systems. The IBC does not include FM's wind-design process (e.g., RoofNav's Ratings Calculator and Assembly Search functions) for determining DWPs. Vague specification language referencing FM may unnecessarily bring the FM wind-design process into play.The IBC also provides 3 test methods for determining wind-uplift capacity of roof systems-UL 580, UL 1897, and FM 4474. It is important to recognize that FM 4474 is a test method used by manufacturers to determine wind-uplift capacity, and FM 4470 is a comprehensive standard covering many aspects of roof system performance. Specifying and only using FM's wind design process in lieu of following the wind-design requirements in IBC, as adopted by local building code, means the minimum legal requirements for wind design technically may not have been met.Understanding the roles that IBC and FM play in the roofing industry is key to understanding the role of the architect or specifier, and the manufacturer when it comes to wind design of roof systems.This blog is for informational purposes only and is not intended to be construed or used as professional design advice. Consult a design professional to ensure the suitability or code compliance of a particular roofing system for any particular structure.
By Authors James R Kirby
Le 16 juillet 2021